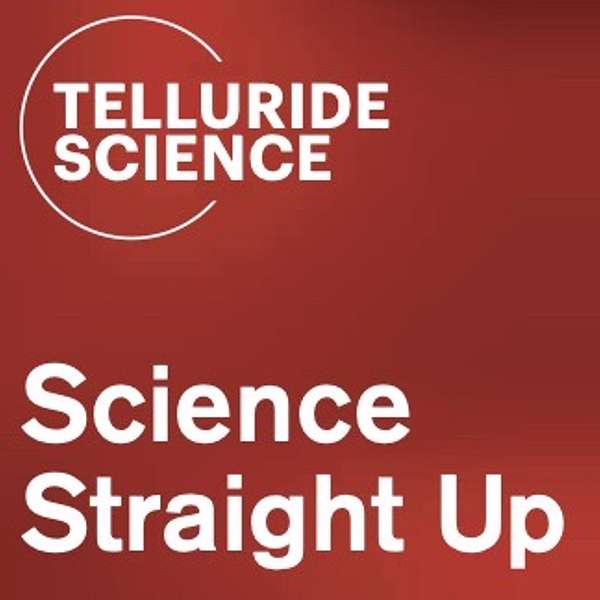
Science Straight Up
In conjunction with Telluride Science, "Science Straight Up" delves into how science impacts our everyday lives. Your hosts, veteran broadcast journalists Judy Muller and George Lewis talk to leading scientists and engineers from around the world.
Science Straight Up
Chromatin: Your DNA in a Package
The double helix structure of DNA discovered in 1953 explained the basic mechanism for how our genetic information can be inherited through replication. But, we've since found that those genes have on/off switches. Understanding how our genes are turned on and off has been complicated partly due to the compacting of DNA with proteins (the histones) into chromatin. Dr. Song Tan has spent years studying chromatin using advanced imaging technology and he and his colleagues have made some big discoveries looking at molecular sized objects. He is joined by Mark Kozak, Executive Director of Telluride Science and by Dr. Richard Kriwacki of St. Jude's Children's Research Hospital. Podcast hosts: veteran broadcast journalists Judy Muller and George Lewis.
Science Straight Up
Season 5, Episode 9
Chromatin: Your DNA in a package—Dr Song Tan, Penn State University
Talk moderators: Richard Kriwacki and Mark Kozak
Podcast hosts: Judy Muller and George Lewis
(Theme music)
(JUDY) From Telluride Science..this is Science Straight Up.
(GEORGE). And, on this episode….
(SONG) We would like to understand how genes are turned on and turned off. Because a lot of human diseases are basically diseases of gene regulation.
(JUDY) Think of DNA as a code…three billion letters long…that acts as a blueprint for life. It’s packed with twenty thousand genes, equipped with on and off switches. We’re all familiar with the double helix structure of DNA discovered over 70 years ago. Stretched out, a strand of it is about six feet long. But, in our bodies. It gets packaged into a very compact form that fits inside our cells. It’s called chromatin, which is made up of pieces called nucleosomes. . Dr. Song Tan of Penn State University has spent years looking into these DNA structures. His Town Talk was recorded before a live audience at the Telluride Mountain Village Conference Center.
(SONG) In every cell in your body, you have about six feet of DNA. Okay? Imagine that every tiny little cell in your body six feet of DNA that has to fit into the nucleus of a cell, which is a fraction of the size of a human hair. So even I have trouble imagining that, and so I've come up this following analogy, which I think is very useful. Fitting six feet of DNA into your cell is equivalent to taking 30 miles of thread and putting it into a basketball. Okay? Do you imagine that thread and you have to fit into a basketball, and you think about, you go, like, I can kind of imagine, I can do it. It's still remarkable. But there has to be some kind of order. It cannot just be just stuffing the thread in the basketball, right? And so, yes, there is order, and this is what your cell does.
(GEORGE) Chromatin is the mixture of DNA and proteins called histones that do that packing job. Dr. Tan helped to organize a workshop on chromatin sponsored by Telluride Science. Each year, leading figures in science gather in Telluride to exchange ideas about cutting-edge research. Dr. Tan has been looking closely at chromatin because he and his team think it could unlock some of the secrets about how genes are switched on and off and how this could help in the battle against cancer and other diseases.
(SONG) Cancer is basically a disease where the gene regulation in a cell has gone wrong. And so again, understanding how genes are turned on and turned off is fundamentally important. Let me use an analogy here. So, think of a city. You have to have goods and services go to from point A to point B at the right time, right place. You need to have traffic lights. You have to control the traffic. You have to regulate the traffic. What happens if there's a blackout or something and the traffic lights go out? Right? You have chaos. Okay? And that's the same thing in a human cell. If you have misregulation, you don't regulate things properly. That often leads to problems, including diseases, including cancer. So that's why gene regulation matters.
(JUDY) Dr Tan and his colleagues use advanced imaging[ST1] methods that can see at the molecular level. And as they study these structures and the hundreds of proteins and enzymes that play traffic cop, they’re learning more and more about the secrets of gene regulation. Their work brought to mind the old sci-fi movie “Fantastic Voyage,” where scientists were shrunk into microscopic size to explore the human body.
(FANTASTIC VOYAGE TRAILER) “If you thought it was too late to discover something entirely new on the screen, Fantastic Voyage will be a stunning experience!
(GEORGE) And Dr. Tan and his team keep finding all sorts of entirely new things on their screens at Penn State.
(SONG) DNA is not just this polymer, long polymer in your body. It has to be packaged. It has to fit into the tiny little nucleus. But that packaging has consequences, and those consequences are important for human health, because again, let me just make this one point, which I forgot to mention, these hundreds of proteins and enzyme I've been telling you about. A lot of them have strong association with human disease, particularly cancers, because they are the master regulators. They are the molecules that control gene expression, Gene regulation, and gene regulation is so important for human health. And because of that, there actually is active work in the field to come up with drugs which can, you know, maybe inhibit some of these enzymes which are too active in cancer cells. So, there's fundamental reasons to understand what's going on in terms of chromatin, but there's also biomedical, human health reasons why we spend so much time investigating this topic.
(JUDY) It was the scientific team of James Watson and Francis Crick who discovered that DNA is structured in a double helix. Now, Dr. Tan and his team[ST2] , have found new structures inside that packaged DNA. And something called the arginine anchor that ties stuff together.
(“Anchors Aweigh” plays).
(JUDY) And inside those microscopic structures, there’s an ideal place to drop anchor: The hot spot.
(SONG) Why is this important? It says that this region over here, that is that these proteins are binding to, is a hot spot. It's a target for these, all these different proteins to do their job they want to interact with that region. We're still trying to understand exactly how important this is and even though I’ve been working in the field for a long time, seeing this just brings up feelings of…it’s remarkable!
(GEORGE) The question-and-answer portion of Dr. Tan’s town talk was led by structural biologist, Dr Richard Kriwacki of St. Jude Children’s Research Hospital and by Mark Kozak, executive director of Telluride Science.
(MARK) I do have a question to begin with, with the arginine anchor, is this part of the protein that's coming in, or is it, is it collected? Does it come in from outside, and what is the relationship between where does it come from?
(SONG) No, this is a good question. So if this is the nucleosome, and we've got different enzymes coming in and doing things, it's part of the enzyme.
(MARIK) Okay.
(SONG) O kay, so think of it as being now, there's many more interactions that happen, okay, but this is often a critical interaction. I kind of think of it as this is this hot spot, and the factors need to make interaction nucleosome to do their job, and it's like they home into that, because there's a rather unique surface on the nucleosome.
(MARK) Can I ask one more question?
(RICHARD) Yes, you can, Mark. (LAUGHTER)
(MARK) Helping sort of us in the lay audience understand with cancer, so my under my basic understanding of cancer is that you often have this somewhat perceived out of control or replication of cells that you don't need that form a tumor, right? How does this enzyme and the nucleosome, what's happening there to in a cancer situation?
(SONG) Yeah, that's a fantastic question, and I'm not sure I can give you the complete answer, okay, because this is, this is exactly what we're still trying to understand the details of, okay, but you can imagine a situation where, in the normal cell, the protein doesn't bind to nucleosome is not active, okay, and that's important. It might have job elsewhere, but you may not want to turn on. And in the cancer cell, you all of a sudden it is being able to turn on. So one example is where you have in your chromosomes, you have breaks in your chromosomes, and you because of those breaks, what happens afterwards is what came from chromosome number five gets attached to chromosome number eight, and it brings together two genes that shouldn't be coming together, and it creates hybrids. It creates a Frankenstein. And that Frankenstein goes off to do horrible things. That's just one example of what can happen.
(JUDY) Dr. Kriwacki joined the questioning.
(RICHARD) I wanted to follow up on Mark's question about the arginine anchor, and I think it may be helpful to the audience to give them some insight into what making the interaction between the enzyme and the nucleosome leads to, in terms of the function that the enzyme performs in simple, in simple, layman's terms.
(SONG)So there's a terrific, tragic but really interesting example that I'm going to describe. It's work from colleagues at University of Washington, Mary-Claire King and Rachel Klevit. They were characterizing a human patient. It was a teenager at the time, the patient had neurological disorders, both cognitive as well as physical, and they did genome sequencing, and they were able to identify a single base change. That was a mutation.
(JUDY) If the name Mary-Claire King sounds familiar, it’s because she’s the geneticist who first identified the BRCA-1 gene that can lead to breast cancer in some women. Her research has radically improved cancer screening and saved a lot of lives. But the case Dr. Tan is talking about involved something else. Here was a neurological disorder that arose from something called the Polycomb protein complex. Researchers are finding that these proteins can remodel the chromatin and cause subtle genetic mutations.
(SONG) And they were able through experiments doing using worms to show that that mutation was causative for the neurological defect, because they found that when they made the mutation in the worm, they got the expected effect. There are relatively few diseases that we know of where a single base mutation causes the disease and we understand the basis for it. The best-known example is sickle cell anemia, another case where you have just one change, one amino acid has changed and it causes disease, but this is now a great example, again, unfortunate, but a really good example of how it just shows. Goes to show how important Polycom is, because that single point mutation turned out to be relatively mild mutation in general, but because Polycom is so important for development, if you had a more severe mutation, there would never have been an organism. So that's an example of you know, how our work, even though we're not, I'm not a physician, I don't see patients. And it was so gratifying when our colleagues told about us about their work, because it really made true all our hopes of providing insight into medicine, into human health through our basic science studies.
(RICHARD) So, I Wanted to ask you about your your vision of the future in in your field, and perhaps beyond, with respect to the tools of structural biology. So can you talk a little bit about you know, what structural biology challenges you'd like to solve in the future, and how improvements in technology may facilitate discoveries in the future.
(SONG) So let me just make sure we define the term structural biology. Okay, so structural biology is very simple. What do the molecules look like? What do molecules in biology look like, Okay, any artists in the audience, there must be some, okay. Have you ever heard the phrase, form determines function? Okay, so in structure, in molecular biology, we have an equivalent. It's basically the same, which is, structure determines function, and function determines structure. So what the molecules look like have strong bearing on what it does, right? And so, why am I structural biologist? I didn't grow up thinking, I want to be a structural biologist. I became a structural biologist. I became a structural biologist because I started working with a very famous structural biologist, Tim Richmond, but then I realized I like looking at the molecules. I like thinking about what the molecules look like, what do they look like, and how does that relate to what it's going to do its function? Okay, so that's that's structural biology. So I mentioned that the work that I showed was done using a method you know, X ray crystallography, you have to crystallize the things you're interested in, and then you shoot X rays through them. More recently, basically everybody has switched over to using a method called cryo electron microscopy, which is basically a really fancy microscope, okay? And we, we can sort of figure out what molecules look like by I want you to imagine that you want to image a car, okay? And the way you're going to image it is you're going to scatter a thousand cars across a large field, and then you're going to go into spacing and to take pictures of it. But the car is not just sitting on its tires. Some of them are flipped around. Some are on the ends. You take enough pictures, you can work out what the three dimensional structure is from all the different views of the car. So there's, this has been a revolution in the last 10, I would say, about 10 years, where, you know, we've got, you know, literally hundreds of these structures, when maybe we had 10 before we've got extra log being able to do a lot faster. And I think there's going to be, you know, just with the existing technology, there's been lots of wonderful discoveries made. I will tell you that we've had our chromatin workshop just for two days, and there's been some remarkable structures, the ones that, you know, I'm just grinning thinking about it, that becomes from from the technology. And I think that's just going to continue. There's other methods. I'm not going to go through details, something we call tomography, which might be even better for looking at things in the cell imaging things in the cell itself. So I think it's really exciting time for gene regulation research and structural biology.
(GEORGE) The audience also joined in the questioning.
(QUESTION FROM AUDIENCE) Really excellent talk and great answers. Just wondering for you, as a structural biologist, if you could comment how artificial intelligence is changing structural biology?
(SONG) Yeah, I'll just give you one example. There is something called the protein folding problem. So your genes, your DNA, they make proteins, and the structural proteins is really important, and I just described to you how difficult it was to get structures right. So I also said that we now have, you know, the atlas of all the genes in your body. And wouldn't it be wonderful if we could predict what the proteins would look like. Because if we could pick what the proteins would look like, we might be able to protect what it's going to do, right? So this is the structure determinants function. For the longest time, the protein folding problem was a unanswered what's the problem that couldn't be solved. It was always five or 10 years away. Okay, so just in the last three to five years now, using artificial intelligence, they have solved the protein folding problem. You can predict, with pretty good accuracy, what a protein is going to look like just given the genetic what the gene, its gene? It's DNA. It's complicated. How it's been done this was it's an organization called DeepMind, funded by Google. It's changed the way we think about things. So in the past, you might say, I'm interested in this protein because it's important for what it's doing. I have to spend five or 10 years to figure out what structure looks like. And then I'm going to ask a lot of questions. Now you can just go look it up and you so, you know, basically you start with, you know, not to say that the prediction is always going to be a hundred percent correct, but it gives you a hypothesis that you can then test, and so it really allows you to leapfrog a lot of technical difficulties to do more interesting experiments. That's just one example of how important artificial intelligence is.
(MARK) Can you explain what the significance of protein folding is? And why do we care about protein folding?
(S0NG) Right. So it gets back to the structure determines function, if you know so protein folding means, given the amino acid sequence, how, what does the protein look like? What's the structure? Okay? So in the past, we had to use experimental techniques to figure it out, because the predictions were not very good. But now, because we, because of what's called alphafold, we, you know, the all the proteins in our body can be have been predicted what the structure looks like.
(QUESTION FROM AUDIENCE) So to go back to the very start of when you were sitting down, and you use the basic analogy of five and eight butting heads and making Frankenstein. So now, does it mean that those of us that are trying to stay healthy and eat well and so forth, it doesn't really matter that much.
(SONG) Oh no, absolutely. It completely matters. Okay? I think that everybody should, should do their best to eat well and stay healthy. Because, look, we're in the early days of, you know, molecular medicine. So think about, you know, the history of medicine. Where are, what are the most important developments in medicine? It's hygiene, okay, just having good, you know, access to running water. Where did the drugs come from? That are a lot of drugs. They were ones which were found by chance. Usually, we're now in the era of Molecular Medicine, where we're trying to figure out the disease comes about because there's some problem with this particular protein. Can we design with the structure in mind? Can we design a drug to maybe, maybe the enzyme is too active in this patient with this mutation? Can we, with the information of what the enzyme looks like? Now, design a drug that's going to work. And I think this is particularly important because a lot of the drugs that exist now, they may work, but then you have side effects. And if you can have one, a drug that works better, so you can use less of it, you might have less side effects. This is the dream. It's coming true. There are definitely, there are drugs that you see advertised on television, which come about from this sort of knowledge, but there's so much more to be done. And so I think that we're still in the early stages of elective medicine, but I'm very optimistic for the future.
(QUESTION FROM AUDIENCE) Part of my question, I was just wondering some of the drugs that you are working on, some of the diseases and some of the drugs that you're working on that have come about by this work.
(SONG) So, that’s a great question. I am what's called a basic scientist. I'm interested in basic questions in biology, but I don't work on the drugs themselves. Okay? I also, from my knowledge of what it takes to do drug discovery, feel that I'm exactly the wrong person to do it, that you need to have an entire lab, really. This is the sort of thing where, actually, in many ways, the drug companies are the right ones to do it. They have the specialized equipment. They're really focused on it. I think if I were to do it, I would be dabbling. I'll be a dilettante, and that's why I'm saying I'm exactly the wrong person to do it. But the information that we gather from our basic science research is being used by industry, okay? And in some ways it's because, you know, particularly the early days of what we were trying to do. It was very, very risky. Nobody had ever done it before. Industry wasn't going to invest in it. And through our early work, we were able to show, hey, this is possible. We can do this sort of thing. And you know, I'm sure that some of our structures that we've done, even though we're not doing drug development on it, is being used. The information is used by industry to do it. And that's the way it should work.
(JUDY) There was some discussion of prions. (PRY-ons)
(GEORGE) You say pryons, I say preeons.
(JUDY) Whatever. They’re proteins folded the wrong way, and can do great damage. They’ve been linked to various forms of dementia and also, mad cow disease.
(SONG) Prions are very interesting in which they can fold in different ways. So one idea that existed was a gene makes a protein and a protein has one structure, okay, it folds up in one way. And the whole idea of a prion is that you can actually fold in different ways. And actually, Richard can talk a lot about because he's an expert on intrinsically disordered proteins, and there's, you know, strong associations between intrinsically disordered proteins and prion type proteins.
(RICHARD) So the basic idea is that all proteins have the potential to adopt a prion structure and to be toxic to cells, and sometimes there are mutations within proteins that will promote that conversion and many such mutations occur in neurodegeneration associated proteins.
(SONG) I'm going to share something so everybody heard about Mad Cow disease, right? So ‘till this day I cannot give blood. Why? Because I was a graduate student in England and in Cambridge when the Mad Cow existed, the disease existed in the animals. And so, because of those two years in Cambridge, and therefore having exposure to it, I'm not allowed to give blood. I'd like to think that if something would have happened, it would have happened by now. (laughter)
(RICHARD) I was gonna see say that there seemed not to be any adverse effects so far. (laughter)
(MARK) Well, I think we should wrap it up on that. Thank you so much. Let's give Dr Tan a round of applause.
(APPLAUSE)
(Anchors aweigh plays again)
(GEORGE) Time to pull up our arginine anchors and sail out of here. We want to thank our sponsors, the Alpine Bank and the Telluride Mountain Village owners’ association. And, a big thank you to Dr. Song Tan for his presentation and to Mark Kozak and Dr. Richard Kriwaki for moderating the session. Our audio engineer for this Town Talk was Dean Rolley assisted by Vicki Phelps. The aforementioned Mark Kozak is Executive Director of Telluride Science and Cindy Fusting is Managing Director.
(JUDY) Annie Carlson runs donor relations and Sara Friedberg is lodging and operations manager. For more information, to hear all our podcasts, and if you want to donate to the cause, go to telluride science-dot-o.r.g. I’m Judy Muller.
(GEORGE) And I’m George Lewis, inviting you to join us next time on Science Straight Up.
[ST1]X-ray crystallography doesn’t see molecules directly. Simpler to just delete “x-ray” here. We also use cryoelectron microscopy (which does see molecules), so leaving it as “advanced imaging methods” is both accurate and and simpler.
[ST2]My lab was the first to determine the structure of a chromatin protein bound to the nucleosome, but the dozens of new structures being determined is the collective efforts of many competing labs around the world.