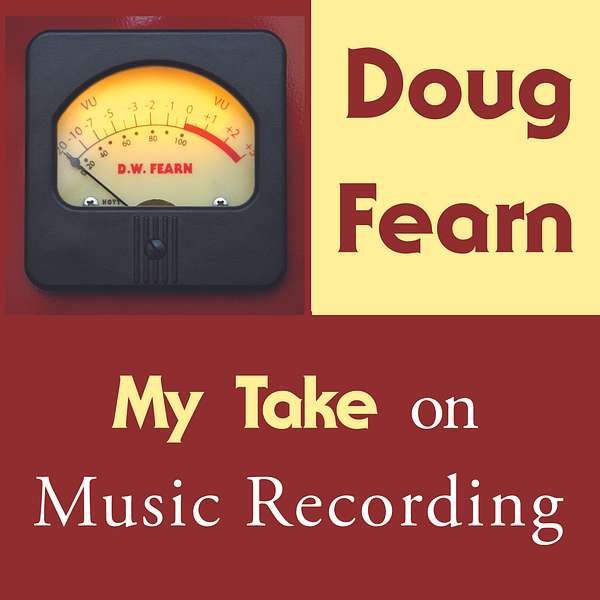
My Take on Music Recording with Doug Fearn
My Take on Music Recording with Doug Fearn
Why Different Gear Sounds Different
What makes one piece of studio electronic gear sound different from another, similar piece?
When it comes to the physical gear we need to record, there are only a few basic categories. You probably use microphones, mic preamps, equalizers, compressors, converters, reverb devices, delays, monitor amps and speakers.
And for each of those categories, there could be dozens of manufacturers, and hundreds of choices. How do you choose one over the other?
And why would they sound different from each other, anyway? They are fulfilling the same function, so you might suppose that the sound of each would be the same.
But they are not the same.
In this episode, I delve into this and explore why things sound different going through different boxes.
Technical Note: This episode was recorded with an AEA KU5A cardioid ribbon mic, through a D.W. Fearn VT-2 mic preamp and VT-7 Compressor. No eq was used. My KU5A is a passive version (the KU5A is normally an active mic). I use a lot of AEA mics in my recording, but except for being friends with founder Wes Dooley, I have no affiliation with the company.
email: dwfearn@dwfearn.com
www.youtube.com/c/DWFearn
https://dwfearn.com/
72 Why Different Studio Gear Sounds Different 25 October 2022
I’m Doug Fearn and this is My Take On Music Recording
What makes one piece of studio electronic gear sound different from another, similar piece?
When it comes to the physical gear we need to record, there are only a few basic categories. You probably use microphones, mic preamps, equalizers, compressors, converters, reverb devices, delays, monitor amps and speakers.
And for each of those categories, there could be dozens of manufacturers, and hundreds of choices. How do you choose one over the other?
And why would they sound different from each other, anyway? They are fulfilling the same function, so you might suppose that the sound of each would be the same.
But they are not the same.
I want to delve into this and explore why things sound different going through different boxes.
We can start with microphones, which are among the most varied of any of the equipment we use. Some variables in a microphone’s sound are obvious, such as the three main technologies used to convert sound waves into electrical signals. We all know that a dynamic mic sounds different from a condenser mic, and a ribbon mic sounds different from either.
As an example, let’s take a large-diaphragm condenser mic, perhaps the most used type in many studios. They all work on the same principle. I will not get into any technical detail here, except to say that all have a capsule, active electronics, and perhaps an output transformer. All have an enclosure to house the parts, and a grille that allows sound through while protecting the fragile capsule from physical harm.
The electronics of a condenser microphone are all essentially similar in design, within the categories of solid-state or vacuum tube electronics.
To be complete, I should say that there is another class of condenser mic, usually called “RF condensers,” which work on an entirely different principle. The Sennheiser MKH line of mics are RF condensers. Their main advantage is that they have less distortion than conventional condenser mics. Otherwise, the same considerations apply to either class of condenser mics.
Oh, and although not much used in the studio, there are also electret condenser mics, which rely on a permanent electrostatic charge on the capsule. Power is still required for the electronics in the mic. Electret mics are what are found in mobile phone mics and other devices that require a cheap and compact microphone.
There is a great deal of variety in the condenser mic capsule design, which accounts for a large part of the differences in sound.
In the electronics, subtle variations occur depending on the exact circuit design, and in the components chosen in that circuit. By the way, the electronics in a condenser mic are not really an amplifier. The purpose of the electronics is to match the extremely high impedance of the capsule to the low impedance we use to convey audio signals. Usually, the tube or transistors in the mic actually have some loss instead of gain. The output level of a condenser capsule is quite high and no amplification is necessary, just the impedance transformation.
Is there a sonic difference between cheap electronic components, good components, and esoteric components? In many cases, yes, although I think the difference between top quality components and esoteric, expensive ones is usually an illusion. For manufacturers building to achieve a specified product cost, using the cheapest parts may be a necessity. Often these parts will sound pretty good, but they may not last as long as quality parts. They may also be inconsistent, which means each mic could sound slightly different, or change over time.
But let’s look at some of the other factors that will make a difference in the sound.
Many condenser mics, especially older designs, have a transformer that converts the intrinsically-unbalanced output of the electronics to the balanced audio we need in the studio. Many recent mics dispense with the transformer and use a solid-state equivalent circuit to achieve the balanced output. Whether this is sonically equivalent, worse, or better than, a transformer is something you have to decide.
Quality transformers are heavy and expensive, and the active-balanced transistor circuit is significantly cheaper and lighter. The active-balanced mic can be made smaller. Personally, I prefer the sound of a good transformer.
What about the “passive” parts of the microphone, such as the case and grille. I think we often underestimate their contribution to the sound of the mic.
The outer shell of the mic might seem to have no effect on the sound, but with some mics, the resonance in that tube of metal can change the frequency response in subtle ways. It can also introduce resonances in the sound, usually in the lower mid-range frequencies. The peak of this coloration can be quite narrow, only affecting a small range of frequencies.
Out in the studio, try tapping on the microphone body and listen for a distinct note. This is easier to hear when the mic is on a stand but not connected. If you can hear anything other than a dull thud, that note may be emphasized in the recordings made with that mic.
This effect can be heard on some dynamic and ribbon mics, too.
Most mic manufacturers take precautions to prevent this resonance, and/or spread it out over a wider range of frequencies. Damping material inside the mic might be used to deaden and broaden the resonance. This material can deteriorate over time and change the sound.
A much bigger contributor to the sound is the grille. That’s the perforated outer cover around the capsule. To see how this affects the sound, we have to imagine what sound waves approaching the mic from different directions will encounter on their way to the diaphragm. Only when the holes or gaps in the grille are aligned with a line from the capsule to the sound source will there be minimal effect on the sound.
Once you move off-axis, the sound probably will see an increasingly-occluded path to the diaphragm. From some directions, the path is entirely blocked, although some sound will leak through by bouncing off the various parts of the grille structure and make their way in.
On-axis, the grille may not make much difference. But as you move off-axis, the sound can be colored in odd ways. I alluded to the off-axis sound anomalies in the previous episode comparing figure-8 ribbon mics with other directional mics. This is a somewhat different effect, but the end result is similar. The off-axis sound is weird, and usually not complementary to the sound of a nearby voice or instrument. Or to the sound of the room, for that matter.
You say you only record things with the mic right in front of the instrument? That might work fine as long as there are no other sounds arriving at the mic from other directions. Even if you are recording one sound at a time, the room sound can sound bad because of this effect. And unless you are recording in an anechoic chamber, there will always be some room sound, for better or worse.
Oh, and the grille can have its own resonances, too, just like the body of the mic. No wonder all these otherwise similar mic designs can sound so different from one another!
Do cables influence the sound? I believe any decent quality cable will neither add nor subtract from the sound of your mics or other gear. Sure, a lousy cable can introduce noise of various kinds, which is an argument for using decent cables. But do you have to spend big bucks on esoteric cables? I don’t think it makes a difference, after listening to lots of these very-expensive versions.
Now we come to the microphone preamplifier, which could be a standalone unit or part of a console, mixer, or converter. I have always believed that the mic preamp is a major contributor to audio quality. After all, if you lose any of the sound coming out of the mic, you can never recover it.
I won’t get into a lot of detail about mic preamps, which I have covered in previous episodes of this podcast, and in videos.
But why would different preamps sound different from each other? The basic function of the mic preamp is to provide 50 to 70dB of clean amplification, to boost the very low level from the microphone up to “line level” that our other gear needs.
Some microphone preamplifiers strive to achieve the “straight piece of wire with gain” goal, with perfectly flat frequency response, low noise, and distortion too low to measure. That would seem to be the ideal mic preamp, would it not?
Without getting into the details, let’s just say that all mic preamps are going to distort when the signal peak is high enough. And those peaks occur a lot, on many instruments and even with voices. How the mic preamp handles those peaks is a large part of how that preamp sounds.
Noise is inevitable in mic preamps. There is a definite limit on how quiet any circuit can be, which is a function of the temperature, all other sources of noise being adequately addressed. Good mic preamps come within a handful of dB of the theoretical absolute minimum of noise at room temperature. The only way to get the noise any lower is to reduce the temperature of the mic preamp circuitry. This is practical for the preamps connected to big antennas for deep space communications. The entire preamp may be bathed in liquid nitrogen, just to get a few dB lower noise level. That can make the difference in hearing or not hearing a spacecraft a billion miles away.
In our world of recorded music, noise from the preamp is rarely a major problem. But to keep the noise acceptable requires very careful attention to the design and circuit layout.
When you have a few musicians, or an entire orchestra, in a room with microphones, just the noise of human activity is usually many dB higher than the noise from the mic preamp. People are constantly moving, even when they are doing their best to keep quiet. The HVAC can be designed for minimal noise, but it is never zero noise. No matter how soundproof the studio is, there can be some very low-level noise from outside.
Does any of this noise matter? I don’t think so, it most situations. A lot of music is intrinsically loud, and it masks a lot of noise that might be heard in recording music that is really quiet.
The character of the electronic noise in our equipment is also a factor. Our hearing is most sensitive around 3kHz, and any noise in this range is much more likely to be heard than noise centered at very low or very high frequencies. A mic preamp designer can make decisions that place less of the noise in the range that we are likely to perceive as annoying.
Distortion is also an issue. In this case, we are talking about harmonic distortion. That distortion generates overtones of the musical notes that can degrade the sound. But it depends on the nature of the overtones. Interestingly, vacuum tubes generally have distortion products that are even-order harmonics of the fundamental note. Solid-state designs, on the other hand, tend to generate odd-order harmonics. Why is that important?
Well, the even harmonics just blend in with the sound, adding some fullness if the distortion is high enough. Most musical instruments are designed to have abundant even-order harmonic overtones.
Odd harmonics are discordant and often add an edge to the sound that most people do not like.
Various design choices in either tube or solid-state gear can have an effect on the degree of even or odd harmonics.
There are other factors in the design that can affect the sound, although generally these are more subtle than the ones I mentioned. Phase shift can affect how “solid” the sound is, especially in the low end. There are other types of distortion as well, most of which are rarely an issue in well-designed gear.
You may notice that I did not talk about frequency response. In any gear designed in the last 50 years, frequency response should never be a problem. Before then, the various ways people consumed music often had limited bandwidth, so designers had little incentive to make the gear with totally flat and extended frequency response. That’s not true today, so poor frequency response is mostly a thing of the past.
However, the frequency response at the very low and very high frequencies can have an effect on the phase response. And that can be audible.
Equalizers are devices that purposely modify the frequency response. Most engineers use equalizers all the time to shape the sound of an instrument or voice, perhaps to make it fit into a mix without conflict with other instruments. Or sometimes to correct for some deficiency in the original sound, or to compensate for defects in other equipment. Eq can also be used to modify a sound and make it something unlike what the source sounds like, for artistic effect.
If there is any piece of gear with high variability in design options, it is the equalizer. The designer has practically an infinite number of choices when designing an equalizer, from the obvious ones like the frequencies chosen and the amount of boost or cut. Another major factor in how an equalizer sounds is the frequency range affected by a particular control. Technically, this is referred to a “Q.” This term was originally defined as “quality” in a radio transmitter or receiver. In that application, you want the radio frequency circuitry to be very narrow, or high Q. That allows a radio receiver to pick out one desired signal and block out all others.
In audio, we use the same term, but in an entirely different context. There is no ideal Q. It depends on what you want to do.
A high-Q cut, for example, might be useful if you want to cut out a discrete frequency that shouldn’t be there. One example, which is largely obsolete these days, is something I discovered when doing live recording with an audience. Back in the 1980s, everyone seemed to have a watch that beeped on the hour. In a large auditorium or concert hall, these beeps could be heard every hour, coming from every direction. Not only that, but no one seemed to have set their watch with precision, so the beeps on the hour might be spread over several minutes.
This is rarely a problem today, since most people use their phones for keeping track of the time.
The beep was designed to get your attention, so it was usually a fairly pure tone at 3kHz, where our hearing is most sensitive. Evidently all the manufacturers use the same internal device to generate this frequency, because every watch was exactly in tune with the others.
To get rid of this annoyance in the recording, I would use a very high-Q cut equalizer, precisely set to 3kHz. It was only engaged for the half-second or so that the beep persisted. The beep would entirely disappear, with negligible effect on the music.
Cutting out all the ringtones people have on their phones is not nearly as easy, although there are software solutions that can do wonders with that type of sound.
So that is an example of a high-Q type of equalizer. Would you use that with music? Probably not, unless it was for a very specialized situation.
If you wanted to create a very piercing sound, a high-Q equalizer set to boost might be just what you want. Or you might want to create the effect of a particularly deficient acoustic space. The high-Q equalizer would allow you to emphasize or cut specific frequencies to emulate the problems in a bad-sounding room.
But in dealing with music, a high-Q boost is probably not going to be useful in most circumstances. A lower Q, which will affect a broader range of frequencies, usually sounds better. Some equalizers give you choices for the Q.
An equalizer can be either peaking or shelving. Peaking means it boosts a center frequency, with decreasing boost as you move away from the peak. That depends on the Q of the equalizer.
A shelving eq starts at some set frequency and boost everything above or below it, depending on whether it is a low shelf or high shelf. This often results in a more natural-sounding modification than a peaking equalizer. This type of eq is only useful for the low-end or high-end of the audio spectrum.
The same factors also apply to cutting frequencies with a shelving equalizer.
The slope of the boost or roll-off also has a major effect on the sound of this type of eq.
There are lots of ways to achieve the frequency modification in an equalizer. Various combinations of resistors, capacitors, and inductors can be used to create a wide range of equalization effects. You can also create the same equalization curves in software.
In theory, all those different approaches to designing an equalizer should sound exactly the same, as long as the various parameters are matched.
But they don’t. With close or similar eq curves, various designs can have very different sonic effects. Some of this has to do with the intrinsic phase shift that occurs in an equalizer. This is an inevitable result of modifying the frequency response, but different designs have very different sounds. Part of good equalizer design is making sure the inevitable phase shift makes sense to our hearing.
Some equalizer designs achieve a zero-phase shift through compensating circuitry. The same can be done in software. This would seem to be a good idea, but to my ear, such an equalizer does not sound at all natural. In the real world, anything that modifies the frequency response, such as sound absorbing material in the studio, causes some phase shift. Out hearing understands this, so it seems believable to us.
You would have to decide for yourself which sound you prefer. That applies to any equalization parameter, not just phase shift. Eq is entirely subjective, which explains the popularity of a great many different equalizers. What works perfectly for one recordist may sound awful to another, and vice-versa. We have to agree that there is no magic equalizer that everyone will prefer.
Equalizers and compressors have been used in recording since the 1930s. Compressors were originally designed to protect radio transmitters from overmodulation, or to prevent excessive groove deviation in disc mastering. Today, we often use a compressor to prevent any signal peak from going over digital zero.
The term “limiter” and “compressor” are often used interchangeably. There is no real definition for either, although most people use the term “limiter” to describe a device that has an absolute maximum level that no amount of signal input can exceed. A “compressor” is a more gentle version.
In the studio, we use compressors to even out the volume of something like a singing voice or bass. Or to increase the overall apparent loudness, either on an individual sound or on the entire mix.
For overload protection, we use a limiter. For that application, a software version works best, since it can “look ahead” and be ready for any peak. Properly designed, a software limiter can do its job perfectly and yet be almost transparent.
There are hundreds of compressor designs in the world, and they all sound different. What makes them different from each other?
Perhaps first we should define some characteristics. Foremost is the amount of compression, usually adjusted by a threshold control.
Many compressors have adjustable attack and release times. The attack time is the amount of time it takes the compressor to react to a sound peak. A slow attack time lets some of the initial sound get through without modification, while a fast attack time will react almost instantaneously to the sound peak. I say “almost” because a compressor cannot react until it receives the audio. Attack times can vary from microseconds, a few millionths of a second, to hundreds of milliseconds. One thousand milliseconds equal a second.
Release time is a measure of how long it takes a compressor to return to nominal gain after it compresses. This can range from milliseconds to several seconds. Some compressors release at a variable rate, depending on how hard the compression was, and for how long.
Another characteristic is the ratio. This is a measure of how much the output increases compared to the uncompressed audio. For example, a 2:1 ratio means that for every 2dB the input goes up, the output goes up 1dB. That’s pretty gentle. A 10:1 ratio is much more dramatic, and that ratio is more in line with our vague definition of a limiter.
A more subtle characteristic is the “knee” of the compression. Picture a graph of the input vs output of a compressor. The curve would be a straight line at 45 degrees with no compression. When compression is engaged, the level follows the linear path up to the threshold point and then it starts to flatten out, indicating that level is being reduced. If there is a sharp break in the graph at the threshold, that might be described as a “hard knee.” The shape of the graph looks like the knee of a person, seen from the side. A “soft knee” is one that has a more gentle transition from linear to compressed.
Those two shapes will sound different, although the difference is not always as obvious as other things you might be able to change on the compressor.
Those are the basic adjustments that affect how a compressor sounds. Of course, the designer will modify the range of these parameters depending on how he wants the compressor to react to sound. All of those design decisions will affect how it works on any given material.
There are several ways to achieve compression. The earliest method was to vary the bias on a vacuum tube to vary the gain of the tube. That requires what are called “variable mu” tubes. They are also sometimes called “remote cutoff” tubes. Both terms describe the same characteristic. The mu of a tube is roughly its gain, so if the tube has variable mu, it has adjustable gain. That characteristic, designed into special-purpose vacuum tubes, provides a way to dynamically vary the gain. A sidechain in the compressor provides a varying DC voltage that determines the gain.
Variable-mu tube compressors have a distinctive sound, much of which is baked into the design of the tube. The response to the level of the audio has some strange anomalies, which contribute to the unique sound of this type of compressor.
To my ear, the sound of a tube compressor follows the dynamics of the music very well, in most circumstances. You can apply a lot of tube compression, for an effect.
An example of a compressor using variable-mu tubes is the Fairchild 660, and many others from that era, often designed for broadcast use. Most of these work pretty much the same way, but design choices gives each model a slightly different sound.
Compressors and limiters using variable-mu tubes were the only common method for decades. This approach has been supplemented with several other approaches. I’ll mention a few of them here, but you should know there are other, less common, approaches.
The variable-mu tube can be somewhat duplicated using a FET, a field-effect transistor. The FET has some characteristics that push it more toward the vacuum tube sound than other solid-state devices like regular transistors and integrated circuits. An integrated circuit can include anywhere from a few to millions of transistors in one compact package.
FET compressors work well, but I was never a fan. They seem to have a hard edge to them that I do not like on most music. They are popular, however.
The best example of an FET compressor is the UREI 1176 and its many clones.
Another approach is to use a light-sensitive photocell, illuminated by a light source, the brightness of which is determined by the signal level. This type of compressor has a different sound, one which many people like for its musicality.
This approach is often called an “optical” compressor.
There aren’t many adjustments on an optical compressor because the characteristics of the light source and photocell define the sound. Since there are thousands of light sources, ranging from incandescent light bulbs to LEDs to fluorescent panels, the options available to a designer are overwhelming. The same applies to the thousands of different photocells available. It is a sad fact that production of these devices depends on demand, and a miniscule industry like professional recording is not large enough to justify continued production. The same may be said of many of the parts used in our favorite vintage gear. Even new designs may gradually change as parts become obsolete and replacements must be found.
Also, the optical elements gradually change over time, so the sound can change depending on the age of the compressor.
The classic optical compressor is the LA2A and its many clones.
A designer can somewhat emulate the characteristics of these various devices used to create a limiter using a solid-state circuit called a voltage-controlled amplifier, or VCA. These were popular in the 1970s, but other technologies have largely eclipsed the VCA over the years. Still, there are engineers that incorporate that sound into their work very successfully. To me, the VCA always adds distortion that I do not like.
An esoteric approach to compression uses a pulse-width modulator, or PWM. This is the same sort of circuit that is commonly used in our homes and industry to control variable speed motors.
The PWM uses an oscillator operating at a very high frequency, ranging from 50kHz to several Mhz. The amount of time the output of this square wave is on or off determines the pulse width. As crazy as it sounds, you can periodically shut off the audio at a rate way above our ability to perceive it. The threshold control varies the ratio between on and off, to set the amount of compression.
This concept goes back to the 1930s (yes, using vacuum tubes) for control of industrial processes that need to have the ability to fine-tune the rate.
Its use as an audio compressor dates back to the 1950s, although the early versions were not particular successful.
I feel that a PWM compressor is the most transparent type of level control we have. I liken the effect to an engineer who has extremely fast hands on a fader, instantaneously adjusting the level as needed. That’s not always what we want, but when it is, the PWM compressors excels.
The PWM compressor introduces almost no distortion in the audio. Most other compressor approaches have an intrinsic distortion that may or may not be objectionable.
Examples of a PWM compressor are the Cranesong STC-8 and the D.W. Fearn VT-7.
Analog-to-digital converters, and their complement, the digital-to-analog converters, should all sound the same, but they don’t. Some of this has to do with the analog input and output stages. Unfortunately, most college engineering programs focus on digital theory and teach almost nothing about analog circuitry. As a consequence, a lot of gear gets designed with very good digital circuitry, but poor analog design. Like a mic preamp, once you lose the audio quality in an analog stage, it can never be recovered.
Better-sounding converters have analog circuits designed by analog experts.
The concept of changing sound from our analog physical world to the digital format we need for recording or manipulation, should be very straightforward. But our hearing is exquisitely sensitive to any modification of the sound that we do not interpret as “natural.” Dozens of factors go into design decisions that make these anomalies more or less evident. Consequently, our converters all tend to sound slightly different. Cost, of course, is a major driver of the quality of the design, although you cannot classify any gear strictly by its price.
I should add the published specifications will never tell you about how a piece of equipment sounds, except in a broad, general way. We do not commonly measure many of the parameters that affect the sound. In some cases, such measurement ability is not even possible.
Our reaction to the sound of any gear is entirely subjective.
That gives you a feel for why different equipment designs we use in the studio may appear to be superficially similar, but sound very different. These principles apply to the other gear we use, such as reverb devices and monitor speakers. Different designs stem from differing goals of the manufacturer.
This gives an enormous palate of sound modifications. That helps us define our individual style. I hope this explanation gives you a bit of insight into why all this gear sounds different. It’s a good thing. Find what sounds best to you and use it. Also, be open to new gear that may initially appear to be far from what you want. An open mind will find lots of variety to help us make compelling recordings.
This has been a simple introduction to why different gear sounds different. If you would like me to discuss any other equipment and how it sounds, or if you would like more depth on a certain topic from this discussion, let me know. If there is sufficient interest, I might expand this to additional episodes covering some of these things in more detail.
Thanks for listening, subscribing, and commenting. You can reach me at dwfearn@dwfearn.com
I’m always looking for ideas for future episodes. This topic was suggested by several listeners.
This is My Take on Music Recording. I’m Doug Fearn. See you next time.